Introduction
The requirements for modern electronic circuits are becoming more and more demanding, including downsizing, the utilisation of high frequency signals, high component densities, and ever-more complicated functionality, Thus, the printed circuit board’s architecture, which serves as the fundamental building block of every electronic circuit, has developed to handle ever-more complicated applications involving signals whose frequency is continually rising. Impedance control, a fundamental operation to guarantee the integrity of the signal, or its correct transmission without experiencing distortions in any operational condition of the circuit, is significant in this context.
PCB traces act like transmission lines with accurate impedance values at each location as the frequency rises. A reflection of the signal is produced if the impedance fluctuates from one point to another as opposed to being constant. The difference in the impedance values directly affects the reflected signal, which travels in the opposite direction of the original signal. Therefore, it is essential to maintain the impedance value as consistent as feasible, which in turn depends on the trace’s width, thickness, dielectric constant (r), thickness, and trace and substrate arrangement on the printed circuit board (PCB). Impedance measurements typically range from 25 to 120.
Impedance analysis
The TDR (Time Domain Reflectometry) measuring technique is typically used to test the impedance value anticipated along the transmission lines and confirm the PCB design. Theoretically, all of a signal’s energy reaches its target as it travels through a transmission line because there is no reflection back towards the source. This can only occur if the output impedance of the signal source is equal to the impedance at each point of the transmission line and the termination line. However, a portion of the signal will unavoidably be reflected if the impedance is not maintained constant.
TDR is a method that uses an oscilloscope and a pulse generator to measure the impedance profile of a device under test (DUT) (Figure 1). Sending a very rapid pulse to the DUT will cause some of it to bounce back toward the oscilloscope, which is being used as a monitoring device, if there is an impedance discontinuity. Once the speed of the signal’s passage through the transmission line is known, the location along the line where the discontinuity occurred may be found by timing how long it takes the reflected signal to return to the oscilloscope. Finally, by contrasting the amplitude of the reflected pulse with that of the original pulse delivered to the DUT, the magnitude of the discontinuity (and therefore the difference in impedance) may be discovered. The TDR approach offers helpful information on potential impedance fluctuations across a large spectrum of frequencies since the transmitted pulse is broadband.

Rule 1 – Which factors should be taken into account for microstrip and stripline?
The dielectric constant, the thickness of the insulating material, the width and thickness of the trace, as well as the PCB’s topmost layers, all affect the microstrip traces’ high characteristic impedance. Based on the operating frequency of the circuit, the insulating material’s dielectric constant should be calculated. Additionally, while working with high frequency signals, the value of the dielectric constant must be kept within the narrowest ranges feasible because it decreases with an increase in dielectric constant (1 GHz or higher). The other factors to take into account, after the dielectric constant, are the traces’ width and thickness. Along with maximum current and maximum temperature change, these variables should also be chosen at the design stage to satisfy this criterion because they have a significant impact on the impedance value. Another important factor that influences the estimation of the characteristic impedance is the thickness of the insulating material. In fact, an impedance change between 5 and 8 can be caused by a 0.025mm increase in laminate thickness. Therefore, it is conceivable to alter the laminate’s thickness during PCB manufacture so as to get the appropriate impedance value while maintaining the same value for the other parameters. Antennas, filters, couplers, and power dividers are just a few examples of high frequency circuits that frequently employ microstrips as a sort of transmission line. It is crucial to maintain direct control over these parameters both during the design phase and during the fabrication in order to ensure the expected impedance values because even a small change in the width or thickness of the trace, in the dielectric constant, or in the thickness of the insulating material can result in a significant change in impedance.
The earliest form of planar transmission line was the stripline, developed by M. Barrett at the Air Force Cambridge Research Center in the 1950s. A planar strip of metal contained between two parallel ground planes generates a stripline, which is seen on the right in Figure 2, and the insulating material of the substrate acts as a dielectric. The characteristic impedance of the stripline is dependent on the width of the conductive strip, the substrate’s thickness, and the relative electrical permittivity of the material. The properties of the dielectric material above and below the conductive trace may change, and the centre conductor does not always have to be equally spaced from the ground planes. Using one of the numerous tools accessible online, or specific software packages that require installation on your PC, you may approximate the impedance values for microstrips and striplines.
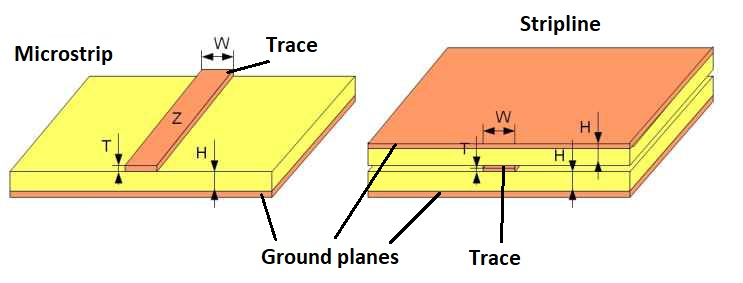
Rule 2: Pick your signals wisely
Designers of printed circuit boards should often explicitly state which signals need impedance management. The component datasheet is quite helpful in this procedure since it often gives comprehensive details on each signal class and the accompanying impedance values. Additionally, it is frequently stated which layer is best for routing individual signals as well as the necessary trace spacing requirements. The clock or data lines of DDR memory, audio and video signals (such as the HDMI interface), gigabit Ethernet communications, or radio frequency (RF) signals are a few examples of signals for which adhering to this rule is fundamentally important.
Therefore, it is crucial that the designer indicate:
- Which signals need impedance management;
- if the signals are single-ended (whose normal impedance values are 40, 50, 55, 60, or 75 ohms) or differential pairs (whose typical impedance values are 100, 90, or 85 ohms).
Rule 3: Keep traces sufficiently apart.
The placement of the different components and other traces on the printed circuit board, as well as the regulated impedance traces, are all of utmost importance. Use a minimum gap of “2W” (better yet, a “3W”), where “W” is the width of the track susceptible to impedance control. This is a widely used yet effective rule of thumb.
In this instance, the spacing between the traces should likewise adhere to the “3W” or “2W” criterion in order to minimise or, if feasible, completely eliminate crosstalk. It should be noted that striplines, which are wrapped in insulating material, are less susceptible to this phenomena since it primarily affects microstrip transmission lines.
The minimum separation should be adjusted to “5W” in the event of high frequency frequencies while maintaining a minimum separation of 30 mils from any other type of signal. This distance should be increased to 50 mils for periodic signals (like clocks) in order to attain a higher level of isolation.
Rule 4: Use vias and bypass capacitors sparingly
Even if the signals are routed symmetrically around them, components and via holes shouldn’t ever be put in between pairs of differential signals. In reality, the impedance value is disrupted by components and through holes, which might affect signal integrity.
Pairs of high-speed differential signals with serial coupling capacitors that are present on the PCB must be arranged symmetrically. Positioning capacitors symmetrically minimises the severity of the signal discontinuity since capacitors cause discontinuities in the impedance value.
Rule 5: Align the trace length
The balancing of the length of the traces helps to prevent that a propagation delay is produced between numerous signals under the supposition that the propagation speed of the signal stays constant in all the traces. This is frequently true for clusters of signals that move quickly together and are anticipated to arrive at their destination simultaneously (or within a tight tolerance). The data lines of a DDR memory serve as an illustration of this.
The length of the traces that make up a pair of differential signals is the same. The delay between the positive and negative signals will be intolerable if length balancing is not done. Practically speaking, a variety of methods are employed to align the length, the most popular of which is to add serpentines to the shorter tracks in order to make up for their relative length to the others (see Figure 3). To lessen the impedance discontinuity, attention must be used while selecting the shape of the serpentine traces.
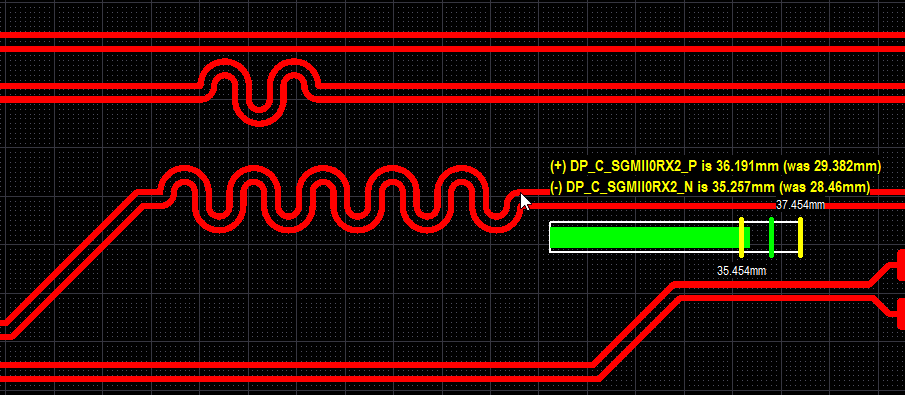